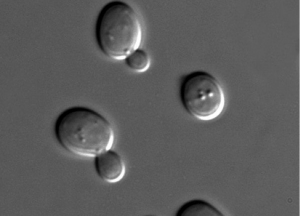
Researches introduced various genetic controllers into S. cerevisiae, a species of yeast, in order to manipulate its cell fate. Source: Wikipedia Commons
A team of researchers from the bioengineering and chemical engineering departments of several Californian universities recently demonstrated a way of ‘reprogramming’ the body’s innate gene circuits using S. cerevisiae budding yeast cells as a model organism. The idea was to interface with existing chemical-based signaling networks between somatic cells in order to divert them to one of 3 ‘cell fates’: mating, nonmating and wild-type. Mating cells always undergo cell cycle arrest; nonmating cells never do and wild type cells may or may not depending upon environmental factors. Cell cycle arrest is a process that dividing cells go through to stop cell division if an environmental anomaly is detected. The ultimate goal of researchers Kate Galloway, Elisa Franco, and Christina Smolke was to establish circuits within the body that can sense aberrant environmental changes and use this information to self-correct corresponding signals.
The reason this research is so pertinent is that many diseases, including one-third of cancers, come from aberrant signals in mitogen-activated protein kinase (MAPK) pathways. Being able to have fine control over this cascade of signals could eliminate the chance of ‘rogue’ cells appearing. Manipulation of signals in this pathway was performed in the study, published in the journal Science, in order to trigger one of the three mentioned cell fates.
A ‘diverter’ in this sense is defined as a biological circuit that forces cells to one of the 3 fates: mating, nonmating or wild type, also known as positive, negative or neutral diversion respectively. One method of diversion was to integrate synthetic and innate circuits, which would provide feedback throughout the MAPK cascade. Synthetic circuits are chemical signaling systems added artificially whereas innate circuits, whilst similar in composition, already exist within the cells. However this method was found to be difficult to implement in complex eukaryotes such as humans due to its potentially destructive actions upon endogenous genes, so an alternative method had to be sought out.
The solution was to build totally separate synthetic circuits that did not act directly on the genetic material of the organism. Instead, they relied upon ‘orthogonal control’ where the body’s existing mechanical and chemical channels remained untouched and were only acted on at fixed control points. The synthetic circuits were composed of RNA based molecules: a regulator, which is responsible for stimulating the type of response (positive or negative); a transducer, which allows signals to be transferred between circuits; and a promoter, which adjusts characteristics of the final output signal such as intensity and frequency. The regulator molecule changed depending upon whether positive or negative diversion was required.
The team overcame several challenges along the way, mainly relating to signal interference between the two diverter circuits, by changing the molecules used as promoters, regulators and transducers. The end result of the project was a dual diverter system that functioned just as efficiently as the two individual diverters, making a real step towards the idea of us being able to modify any of our own ‘badly behaved’ genetic circuits. The real problem now is scaling the system up from a unicellular to a multicellular organism, a challenge that faces many such projects today.
References:
Kate E. Galloway et al. Dynamically Reshaping Signaling Networks to Program Cell Fate via Genetic Controllers. Science 341, (2013).