The inner core: a mystery right below our noses
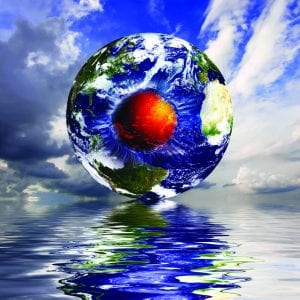
Journey to the Center of the Earth
While many fictional tales have been written about worlds beneath our familiar soils, research regarding the core of the Earth has only begun to scratch the surface. With temperatures around 6,000 K and pressures above 330 GPa, the inner core is an inhospitable place (1). It has only been a hundred years or so since scientists determined that the Earth has an inner core. Nonetheless, for thousands of years mankind has benefited from one of the inner core’s byproducts, its magnetic field, in both navigation and protection from solar winds. Although researchers now understand how the magnetic field works, much more remains to be discovered about the history and peculiarities of the core.
Early History
Edmond Halley first proposed the notion of an inner core in 1686 (2). He theorized that an inner globe that rotated separately from the rest of the Earth existed within the shell of the Earth’s crust. The inner globe’s magnetism would provide a magnetic field for the entire Earth (2). Halley’s “Hollow Earth” model was beyond testability at the time, as it is to this day impossible to drill to the center of Earth.
Waves and Layers
During earthquakes and their aftershocks, shockwaves are not only sent out to the surface, but also below into the Earth. If earthquakes are powerful enough, some of these waves, known as body waves, may bounce to places on the other side of the planet, where seismological instrument can detect them (2, 3). In the early 20th century, Beno Gutenberg noticed that there were zones where these compressional waves did not appear, suggesting that a fluid core prevented their passage or diverted them. The patterns also suggested that the Earth was not filled with air underneath, as Halley had first suggested. In 1936, Inge Lehmann noticed that some of these compressional waves were in these “shadow zones” of the liquid core (2, 3). Given that waves move faster in solids than in liquids, Lehmann hypothesized that a solid core within a larger liquid core would account for the observed deviations in the waves’ trajectories (2). In 1952, Birch suggested that the pressure within the Earth could be high enough to solidify some of the iron within the Earth into a solid inner core (3). Analyses of another form of seismological data, whole-Earth oscillations, provided further evidence that there is a solid inner core, as these larger oscillations carry information about density (2, 3).
Since these discoveries, scientists have found that earthquakes emit many different kinds of body waves. These waves have frequencies between 0.5 and 1.5 Hz and can carry information about the physical structure of the inner core and the inner-outer core boundary (3). In terms of notation, “P” denotes a primary wave, “S” a secondary wave, “K” passage through the outer core, “I” passage through the inner core, “i” reflection off of the inner-outer core boundary, and “J” the conversion from a compressional wave to a shear wave (3, 4). The fastest waves are primary (P) waves, which pass through the mantle but are either reflected or diffracted by the outer core (4). P waves are compressional waves, which travel via a series of compressions and rarefactions. On the other hand, secondary (S) waves are slightly slower and are shear waves, which move up and down perpendicular to their direction of travel. There are also many variations of P waves. For example, PKP waves can penetrate the outer core, but not the inner core. Both PKIKP and PKJKP waves pass through both the outer and inner core, while PKiKP waves bounce off the surface of the inner-outer core boundary (3, 4).
PKiKP waves indicate that the inner core has a radius of 1220 to 1230 km (2). Some of these waves also suggest that there is an intermediate region of both solid and liquid metals around the inner core believed to be less than 10 km thick (2).
PKJKP waves pass through the outer core as compressional waves before becoming shear waves through the inner core and reverting to compressional waves as they pass back out (3). The conversion from compressional waves to shear waves results in energy loss, making it difficult to detect these waves. The presence of these PKJKP waves was discovered in the early 2000s as the last piece of evidence needed to determine that the Earth contains a solid inner core surrounded by a liquid outer core (3).
The Core’s Oddities
At first it was believed that the boundary between the outer and inner core was spherical. In the 1980s, however, analyses of PKIKP waves revealed that the core may have a prolate shape, where the core would be elongated like a football (2). PKiKP waves have also indicated that the topography of the inner core may not be totally smooth (3).
Another aspect of Halley’s now debunked theory was that the inner core could rotate separately from the rest of the Earth. PKIKP waves picked up in Australia in the 1980s suggested that the core does rotate at a different rate. Adding to these findings, seismographs in Alaska caught another set of waves produced in the South Sandwich Islands that indicate superrotation, or that the core rotated faster than the rest of the crust and mantle of the Earth. Later analyses differ regarding how much faster the core’s rotation is, ranging from 0.3 to three degrees per year (2, 3). Other estimates put the superrotation at 0.1 to one degree every million years, prompting some to argue that there may not be any superrotation at all (3).
Analyses of the same data from the South Sandwich Islands have caused speculation that the inner core is not a uniform sphere of iron. On average, the inner core seems to be growing one millimeter annually. However, temperature variations in the mantle may cause regional differences in growth. Colder regions of the mantle, such as those along the East Pacific and the eastern Indian Ocean, would cool the outer core faster, allowing for more growth of the inner core below; the corresponding upper layers of the inner core also transmit waves faster in this region. The mantle under Africa and the Pacific is known to be warmer, thereby slowing growth. (2).
As mentioned earlier, just as there are variations in growth rate, there are also variations in the speed at which body waves cross the inner core. Waves moving in the north-south direction generally travel two percent faster than those in the east-west direction (3, 5). Anisotropy is the term used to describe a situation where a property, like wave speed, varies with direction (5). In the core, anisotropy is correlated to the degree of alignment of iron crystals (2). In contrast, some parts of the core are isotropic, meaning that waves’ speeds do not vary with direction as they travel through the core; this trend presumably results from less crystal alignment. Isotropic regions include the upper 100 to 250 km of the western inner core, the upper 400 km of the eastern regions, and the polar regions (3, 5). Below these depths, there is strong anisotropy, far more prominent in the Western Hemisphere than in the Eastern (3, 5).
The magnetic field could align the iron crystals of the inner core, causing the anisotropy seen in body waves, although some experts believe that the field is not strong enough to do this (3). Another possibility is that the alignment of the iron crystals may have occurred via convection in the inner core itself that happened early in its history, which would explain the anisotropy deeper within the core. To explain the isotropy by the poles, scientists speculate that the inner core grows larger mostly by the equator, causing a radial anisotropy that would appear isotropic as waves travel through the core (3).
Models have been proposed to explain the hemispheric differences in anisotropy. For example, the translation model has the core melting in the east and crystallizing in the west. However, this would require convection to happen in the inner core, which is unlikely (3). The model also struggles to explain the sharp division between hemispheres. The thermochemical flow model proposes variations in the magnetic field such that a concentrated field under Asia would cause solidification in the East, allowing for random crystal formation and less anisotropy. However, this theory does not explain why the deeper layers are anisotropic, while the upper layers remain isotropic (3).
An Inner-Inner Core?
While previous studies failed to provide solid evidence that the inner core itself has any layers, a new study from the University of Illinois and Nanjing University found that there appears to be an inner-inner core, with a radius half that of the entire inner core. This inner layer has iron crystals aligned north-south, while the outer layer has crystals aligned east-west. This alignment may explain why waves that pass through the outer parts of the inner core travel more quickly in the north-south direction, as seen in previous studies. The different orientation in the inner-inner core allows it to have a fast axis aligned east-west along Central America and Southeast Asia (5). The new study suggests that the two layers are made of different crystal types or possibly different phases of iron (6).
The study used a new type of analysis to come to their conclusions. Instead of evaluating the waves produced by earthquakes, the researchers analyzed the echoes from large earthquakes known as coda waves. These echoes are often treated as background noise, but, by enhancing and matching their signals, researchers separated the two different fast corridors in the outer and inner parts of the inner core. Scientists are not sure why the inner core is divided as such; some speculate that the separation of the two layers may coincide with the emergence of an equatorial component of the magnetic field some 600 million years ago (5, 7).
The Magnetic Field
The core is made of iron, a paramagnetic metal. At the temperature and pressure of the inner core, the iron would likely crystallize to form a hexagonal, close-packed pattern, but, in the outer core, the iron would remain a conductive fluid (3). The magnetic field arises from convection in the outer core; liquid iron alloy rises due to heat before it descends as it cools by contact with the mantle (8). This process yields an electric current that can create a magnetic field (5).
Analyses of rocks around the world seem to imply that the solid inner core was not always present. Recent findings indicate that the inner core may have only formed about one to 1.5 billion years ago (8). Geological records indicate that the strength of the magnetic field decreased over a period extending between 2.5 billion and 1.3 billion years ago before increasing suddenly. Researchers believe that reduced convection due to cooling of the iron core caused the decline, but that a later nucleation event created a solid inner core, causing the sudden increase in magnetic field strength (9). Inner core formation would enable more efficient convection, as lighter nonmetals would end up in the molten outer core and promote buoyancy (8). Additionally, the freezing of the inner core releases heat, promoting further convection and maintaining the magnetic field (3). This model predicts a slower decay in field strength over time, which seems to be the case according to an analysis of rocks from the past 200 million years (9). This model also predicts the one millimeter annual growth rate seen in other studies (8).
Although much of this model makes sense, more data, especially from older rock samples, would help verify its claims. The slower-than-expected rate of decay is quite a positive finding. The Earth’s neighboring planet Mars had a strong magnetic field early on, but it only lasted for half a billion years (8). Its decline is likely related to Mars’ smaller size and inability to effectively maintain processes like convection and heat production that keep the Earth’s magnetic field strong.
Future Research
While technology has come a long way since the times of Halley and Lehmann, there are still gaps in data collection. Unfortunately, most of the subduction zones where earthquakes occur lie near the equator, limiting researchers’ ability to study the core spatially. During the 1990s, rarer earthquakes in the South Sandwich Islands expanded our understanding of the inner core, but, even these recordings are not enough, as waves from these islands only sample the Western Hemisphere (2, 3). Data collection is also limited by the fact that most of the world is covered by oceans, although a few seismographs have been placed underwater (2). Additionally, the landmasses, as well as the wealth of countries, are not evenly distributed. Along with more data collection comes the need for novel methods of analyzing seismological data, such as those used in the inner-inner core study. Another area for future development is the investigation of PKJKP waves, which often reach the surface with little energy remaining (3). Claims of these waves’ existence have often been met with skepticism, but a more systematic way of detecting these waves could reveal more about the core (2).
Many mysteries regarding the core remain, with even more theories abounding about how the core gained its anisotropic properties. The new finding of the inner-inner core raises as many questions as it answers, especially about how the core came to its present state. Studying the core could reveal how the Earth developed and how it came to have the magnetic field essential for life on Earth.
References:
1. Anzellini, S., Dewaele, A., Mezouar, M., Loubeyre, P., & Morard, G. (2013). Melting of Iron at Earth’s Inner Core Boundary Based on Fast X-ray Diffraction. Science, 340(6131), 464-466. doi: 10.1126/science.1233514
2. Tkalˇci´c, H. (2015). Complex inner core of the Earth: The last frontier of global seismology. Reviews of Geophysics, 53(1), 59-94. doi: 10.1002/2014RG000469
3. Deuss, A. (2014). Heterogeneity and Anisotropy of Earth’s Inner Core. Annual Review of Earth and Planetary Sciences, 42, 103-126. doi: 10.1146/annurev-earth-060313-054658
4. Storchak, D. A., Schweitzer, J., & Bormann, P. (2003). The IASPEI Standard Seismic Phase List. Seismological Research Letters, 74(6), 761-772.
5. Song, X. (2015, December 11). The Core Within Earth’s Inner Core: Year in Review 2015. Retrieved from http://www.britannica.com/topic/Core-Within-Earths-Inner-Core-The-2046720
6. Ahlberg, L. (2015, February 9). Earth’s surprise inside: Geologists unlock mysteries of the planet’s inner core. Retrieved from https://news.illinois.edu/news/15/0209innercore_xiaodongsong.html
7. Morelle, R. (2015, February 10). Heart of Earth’s inner core revealed. Retrieved from http://www.bbc.com/news/science-environment-31322817
8. Study indicates Earth’s inner core was formed 1-1.5bn years ago. (2015, October 7). Retrieved from https://news.liverpool.ac.uk/2015/10/07/research-indicates-Earths-inner-core-was-formed-1-1-5-billion-years-ago/
9. Biggin, A. J., Piispa, E. J., Pesonen, L. J., Holme, R., Paterson, G. A., Veikkolainen, T., & Tauxe, L. (2015). Palaeomagnetic field intensity variations suggest Mesoproterozoic inner-core nucleation. Nature, 526(7572), 245-248. doi:10.1038/nature15523