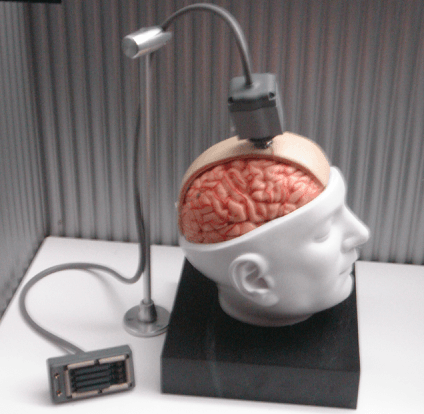
Modern electrical engineers and neuroscientists join forces to develop brain-computer interfaces that improve the lives of patients with neurodegenerative diseases. (Source: Wikimedia Commons)
Whenever we perform a complex motion, our brain and muscles work together effortlessly to execute the desired movements with accuracy and precision. However, patients with spinal cord injuries or neurodegenerative diseases can no longer perform these once-easy tasks because the connections between their brain and muscles are damaged. To improve these patients’ lives, biomedical engineers have sought to restore patients’ motor functions with brain-controlled prostheses, artificial devices that bypass the damaged neural tracts. These devices monitor electrical activity in the brain and deliver thought commands to prostheses like computer cursors and virtual keypads (1).
So far, these devices have remained imprecise because of the brain’s remarkable complexity. In the human brain, millions of neurons, the biological “switches” that make up the nervous system, fire in complex patterns to generate our thoughts and actions. However, current brain-controlled prostheses are only able to obtain information from a few hundred neurons, so these devices’ actions can be slow and inexact because of their limited sampling abilities (1).
Now, an interdisciplinary team of engineers and scientists led by Krishna Shenoy, professor of Electrical Engineering, Bioengineering, and Neurobiology at Stanford University, has developed a novel algorithm to reduce errors in brain-controlled prostheses (1). To create their algorithm, the team needed a better understanding of “brain dynamics,” or the “rules that characterize the interactions of the millions of neurons that control motions,” as defined by Jonathan Kao, first author of the Nature Communications paper on the research (2). To understand “brain dynamics,” the researchers examined how monkeys naturally perform arm movements. The team conducted hundreds of experiments in which monkeys used their arms, hands, and fingers to reach for targets on a video screen, while the researchers observed what the 100-200 neuron samples looked like during the monkey’s actions (1). These experiments helped reveal the “brain dynamics” underlying arm movement.
Then, the team used this new information to create an algorithm that could analyze the prostheses’ small sample sizes and make corrections so that the small samples’ dynamics were more representative of the whole animal’s brain dynamics (1). These small corrections were intended to make the thought-controlled prosthetics more precise. To test their algorithm, the researchers trained monkeys to identify targets on a keypad. The keypad consisted of several rows and columns of circles. When a light flashed on a particular circle, the monkeys were trained to reach for that circle with their fingers (1).
To set a baseline for their experiment, the researchers determined how many targets the monkeys could correctly tap with their fingers in 30 seconds. The monkeys averaged 29 taps in 30 seconds (1). Then, the researchers conducted the real experiment, in which they only counted taps that came from the monkeys’ thought-controlled computer cursor. Although the monkey may have continued to move his fingers, only hits from the thought-controlled prosthetic were counted. The prosthetic scored 26 taps in 30 seconds, 90 percent as accurate as the monkey’s fingers (1). For comparison, members of the Shenoy lab also tested other prostheses that use alternative algorithms to decrease sampling error. Out of these alternatives, the most accurate device scored 23 taps in 30 seconds (1).
Members of the Shenoy lab hope that their algorithm will improve the lives of patients with paralysis and amyotrophic lateral sclerosis (ALS, also called Lou Gehrig’s Disease). ALS slowly degrades one’s ability to move. Today, ALS patients may use an eye-tracking system or a “head-mouse,” which tracks head movements, to direct computer cursors (1). Both of these technologies fatigue the user, and neither provides the intuitive and natural control of thought-directed devices. According to Shenoy, his algorithm can be “further refined and optimized to give brain-controlled prosthesis greater performance, and therefore greater clinical viability” (1). Looking forward, the Shenoy Lab hopes to conduct a pilot clinical trial of their thought-controlled cursor on human patients with spinal cord injuries.
References:
- Stanford School of Engineering. (2015, July 31). Brain-controlled Prosthesis Nearly as Good as One-finger Typing. Retrieved August 9, 2015, from http://www.sciencemag.org/content/348/6237/858.full
- Kao et al. (2015, July 29). Single-trial dynamics of motor cortex and their applications to brain-machine interfaces. Retrived August 9, 2015, from http://www.nature.com/ncomms/2015/150729/ncomms8759/full/ncomms8759.html